ADVANCED NEUROMONITORING – A BOON IN CLINICAL PRACTICE
Dr. Shilpa Nagmoti Jaiswal
MD.,DNB., DM Neuroanaesthesia and Neurocritical care., PDCC., MNAMS.
Assistant Professor, Department of Anesthesia, SSH, Nagpur.

Introduction– In Anaesthesia practice, it is important to continuously monitor the organ of interest. Brain is an organ of organs and very complex one to monitor. With so many independent structures in CNS, it is impossible to monitor all of them continuously. Thus, the neuromonitoring can be a challenging task and the information gained may not always transform into clinical utility. However, the field of neuromonitoring has changed remarkably in recent years and in many of the cases neuromonitoring is even considered the standard of care. Thus, neuromonitoring is, for the most part, “surveillance” to improve patient safety and optimize patient outcomes.
What is the need to monitor ?
Neuromonitoring is crucial to detect early signs of neurological dysfunction during surgery or critical illness and allows us to intervene promptly and prevent irreversible CNS damage. It identifies potential issues like nerve injury, ischemia, spinal cord compression etc. and helps improve patient safety and outcomes and minimise neurological complications. It also provides real time feedback to guide treatment decisions and monitor the effectiveness of interventions . Neuromonitoring thus helps in early detection of potential damage, enhances surgical safety, helps us give personalised care to patients and also provides us with prognostic information.
What all can be monitored ?
- Cerebral blood flow
- Intracranial pressure
- Evoked potentials
- Cerebral oxygenation
CEREBRAL BLOOD FLOW MONITORING –
Cerebral blood flow (CBF) is critically important for brain function and viability. Hypoperfusion can cause brain damage through ischemic injury whereas hyperperfusion can cause the breakdown of the blood–brain barrier and can cause seizures, headaches, encephalopathy, and ischemic or hemorrhagic stroke.

Transcranial Doppler (TCD) which uses a principle of Doppler sound waves, is the most commonly used technique to measure the cerebral blood flow (CBF) and cerebral autoregulation. A 2 MHz probe (Figure 1) is used transpterionally, Transorbitally or subocciptally site is also used to insonate cranial vessels.
Transcranial sonography, thermal diffusion flowmetry, laser doppler flowmetry are other monitoring techniques used to monitor CBF.
Monitoring CBF and autoregulation is useful in Subarachnoid haemorrhage, carotid endarterectomy to detect ischemia or emboli, head injury, Cardiac surgery, Hepatic encephalopathy and Eclampsia.
INTRACRANIAL PRESSURE MONITORING –
It is defined as the pressure within cranial cavity relative to the atmospheric pressure. Normal ICP is 5–15mmHg in adults; 3–7mmHg (children) and 1–5mmHg (infants). Cut off values for treating ICP depends on the intracranial pathology. For head injury, treatment is initiated when the ICP exceeds 20–25 mm Hg.
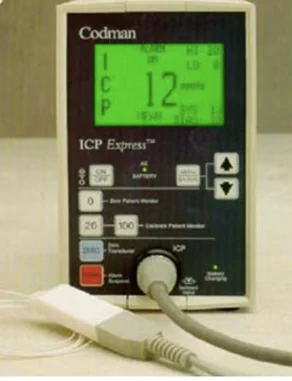
ICP can be measured invasively using Fluid-filled external pressure transducer, Miniature strain gauge transducer (Codman)(figure 2), Fiberoptic (Camino), Spielberg ICP system or noninvasively using Tympanic membrane displacement, TCD and Optic nerve sheath diameter.
ICP Monitoring is indicated in
- Severe head injury with abnormal CT head scan
- Severe head injury with normal head CT with any of the two—age >40 years, systolic blood pressure <90 mm Hg, abnormal motor posturing
- Systemic diseases causing raised ICP- Rye’s syndrome, hepatic failure
- SAH, Malignant cerebral infarction, meningitis
EVOKED POTENTIAL MONITORING –
Evoked potentials are recordings of electrical activity of specific neuronal pathways in response to external stimulus. When neural tissues are stimulated, the electrical activity ascends along specific neuronal pathway. Based on stimulation site and recording location, characteristic waveform of the traveling impulses can be recorded. The recordings involve either the sensory pathway-SSEPs or motor pathway- MEPs.
Somatosensory Evoked Potentials (SSEP)
It denotes the electrical activity recorded along the sensory pathways via the dorsal column in the spinal cord and the scalp overlying the sensory cortex (Figure 3). Latency prolongation by ≥10% from the baseline or decrease of amplitude by ≥50% is considered significant. SSEPs are used for spinal procedures like intramedullary tumors, scoliosis surgery, spine stabilization and for cerebral vascular surgeries like Intracranial aneurysm, CEA, also to identify position related nerve injuries.

Brain Stem Auditory Evoked Potential is the recording of the activities of the auditory pathways following stimulation of the cochlear nerve. The electrical impulses travel along the vestibulocochlear nerve to the brain stem, midbrain and reach the primary auditory cortex. BAEP is used in cerebellopontine angle surgeries , Brain stem procedures and posterior circulation vascular surgeries.
Visual Evoked Potential (VEP) records the electrical activity from neural generators along the visual pathway.
MOTOR EVOKED POTENTIALS
Following stimulation (electrical or magnetic) of the motor cortex, electrical impulses travel along the cortical spinal tract, descend at the level of brain stem, travel down to the anterior funiculi of the spinal cord, and result in muscle activity. The dictum is that unchanged stimulus parameters should produce similar responses in a particular group of muscles so, changes are considered significant when there is: an increased requirement of stimulus strength (>50 V) to produce the same initial response OR increased number of stimuli to achieve the same initial response OR decrease in amplitude by more than 80%.
MEP is used For spinal procedures as a supplement to SSEP, e.g., scoliosis correction surgery, intramedullary tumors, Spinal decompression procedures, Intracranial tumors involving motor cortex and is also used for mapping of motor cortex.
CEREBRAL OXYGENATION MONITORING
The purpose of monitoring cerebral oxygenation is to identify ischemia at an early (reversible) stage, so that interventions can be planned. Monitors of cerebral oxygenation include jugular venous oximetry, cerebral oximetry, and brain tissue oxygen.
Jugular venous oximetry (SjvO2) measures the oxygen saturation of the venous blood at jugular bulb(figure 4 ). SjvO2 represents the global oxygen extraction of the cerebral tissues. Cerebral oxygen consumption (CMRO2) is calculated as a product of CBF and arteriovenous oxygen difference (A-VO2). SjvO2 is indicated inhead injury patients to identify secondary neuronal injury, SAH patients, to differentiate vasospasm from hyperemia and to titrate hyperventilation therapy.
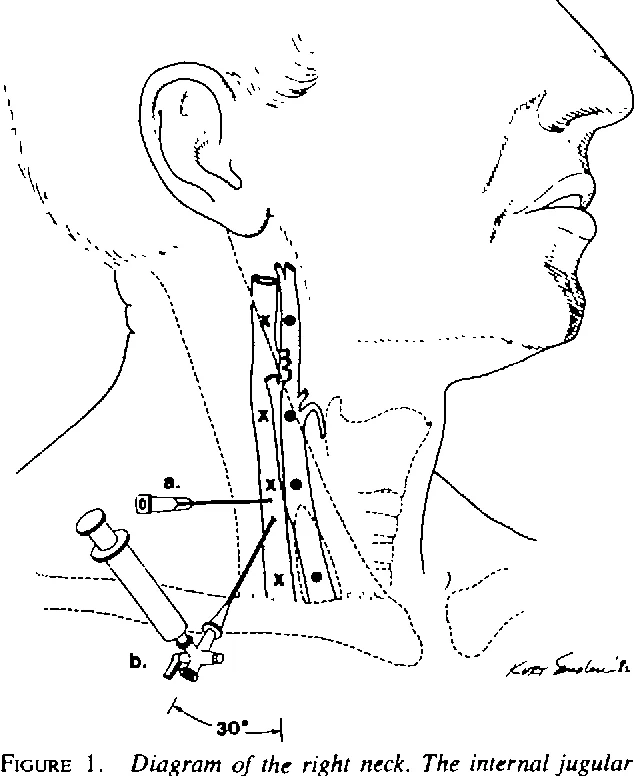
Regional cerebral oximetry– Cerebral oximetry noninvasively and continuously measures the regional cerebral tissue oxygen saturation (rSO2) by near-infrared (NIR) spectroscopic technique. Principle behind the NIR technology is based on the Lambert Beer equation. ITt can be used in surgeries such as CEA, cardiac surgeries, neuroradiology suite during balloon occlusion testing of ICA, ICU, to detect cerebral vasospasm, etc.
Brain tissue oxygen monitoring is another method to monitor cerebral oxygenation.This technique measures (focal) oxygen tension at the brain’s interstitial space. It represents the oxygen available for mitochondrial oxidative phosphorylation. Brain tissue oxygen monitoring (PbtO2) is determined by the CBF and the difference in cerebral arterial and venous oxygen tension. PbtO2 can be measured by two different techniques- Modified Clark electrode and Optical fluorescence. PbtO2 monitoring is useful to titrate individualized therapy such as osmotherapy, hyperventilation, CPP, patient positioning, and deciding on timing of decompressive craniectomy.
Cerebral microdialysis – This monitoring modality measures the brain’s metabolic substrate, both aerobic and anaerobic, in the interstitial tissue space. The probe consists of a coaxial double lumen tube covered by a dialysis membrane. The tube is continuously infused with an artificial CSF using a microinjector pump at a rate 0.3 μl/min. Depending on the concentration gradients and the pore size of membrane, metabolic substrates diffuse into the probe and are collected in to a sampling bottle. The sample is then analysed.
In ischemia, there is accumulation of lactate and increase in lactate/pyruvate ratio (>25, significant) and fall in glucose <0.8 mmol/L (critical). Glutamate increase during ischemia indicates excitotoxicity. CMD helps in monitoring metabolic substrates to pick up neuronal ischemia at a reversible stage, e.g., in severe head injury, SAH and can also help us guide glucose therapy in neuro ICUs.
Conclusion Any one modality of monitoring is very unlikely to give complete picture of the cerebral functioning. At best it can give complete information on one aspect of the cerebral functioning. Therefore, it is essential to have multimodal monitoring for decision making in neurological cases. This has been made possible by advanced neuromonitoring and so it is indeed a boon to perioperative physicians.